X-ray CRYSTALLOGRAPHY is about to become a history, and CRYO-ELECTRON MICROSCOPY has revolutionized the hidden mechanism of intracellular cells.
In the basement of a building in Cambridge, a technological revolution is brewing.
A cumbersome, approximately 3 meter high metal box transmits quietly terabytes of data through an orange cable that connects the cells. This is one of the most advanced cryo-electron microscopes in the world: cryo-electron microscopy images frozen biomolecules by electron beam to obtain the three-dimensional structure of the molecule. Standing next to the $7.7 million instrument, Sjors Scheres, a structural biologist at the UK Medical Research Council Laboratory of Molecular Biology (LMB), said that cryo-electron microscopy is very sensitive and shouts It will bring great errors and lead to experimental failure. “The UK needs more cryo-electron microscopy because it will become the mainstream of structural biology in the future.â€
Low temperature electron microscopy shocked structural biology. In the past 30 years, cryo-electron microscopy revealed the fine structure of ribosomes, membrane proteins and other key cellular proteins. These findings are published in top magazines. Structural biologists say it's no exaggeration to say that cryo-electron microscopy is revolutionary: low-temperature electron microscopy can quickly generate high-resolution molecular models, far beyond X-ray crystal diffraction. Labs that rely on the old method to win the Nobel Prize are also working hard to learn this technology. This new model accurately reveals the necessary mechanisms for cell manipulation and how to target disease-associated proteins.
“Cryogenic electron microscopy can solve many puzzles that could not be solved before,†says David Agard, a structural biologist at the University of California in San Francisco.
A few years ago, Scheres was recruited into LMB with the task of helping to improve the cryo-electron microscope, and he finally succeeded. Last month, they published the most exciting achievement in this field: a high-resolution picture of Alzheimer's-related enzymes, including 1200 or so amino acids of the enzyme with a resolution of a few tenths of a nanometer.
Biologists are still working to develop the technology to solve the subtle structure of small or deformable molecules – a challenge for cryogenic electron microscopy. Eva Nogales, a structural biologist from the University of California, said that it is a revolution or a leap. A cryo-electron microscope does open a door.
Protein crystallization
There is an unwritten view in the field of structural biology: structure determines function. Only by knowing the atomic arrangement of biomolecules can researchers understand the function of this protein. For example, how ribosomes make proteins based on the sequence of mRNA, how the molecular pores are opened and closed. For decades, the analysis of protein structure has an innocent king, X-ray crystal diffraction. In X-ray crystal diffraction, scientists crystallize proteins, then X-rays, and then reconstruct the structure of the protein based on X-ray diffraction. In the more than 100,000 protein terms of the Protein Data Bank, more than 90% of the protein structure is resolved using X-ray crystallography. Many Nobel Prizes are also associated with this technology, such as the 1962 Nobel Prize in revealing the DNA double-stranded helix.
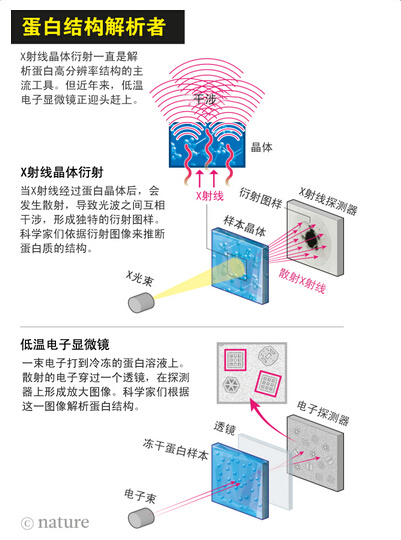
Although X-ray crystal diffraction has always been the best tool for structural biologists, it has significant limitations. Scientists may take years to find ways to form large crystals of protein. Many basic protein molecules, such as proteins embedded in cell membranes, or proteins that form complexes, cannot be crystallized.
When Richard Henderson came to LMB in 1973 to study the structure of the rhodopsin, a protein that uses light to transport protons into the membrane, X-ray crystallography is the tool of choice. Henderson and his colleague Nigel Unwin succeeded in making two-dimensional crystals of the protein, but not for X-ray diffraction. So they decided to use an electron microscope.
At the time, electron microscopy was mainly used to study viruses or tissue sections stained with heavy metals. A beam of photons is struck on the sample, and new electrons are detected and used to resolve the sample structure. This method succeeded in producing a subtle picture of the first virus, a tobacco virus. But the staining makes it impossible to see the individual proteins, let alone the atomic details. Agarad said that the sample is either full of spots or not stained, you can only see the outline of the molecule.
Herderson et al. omitted the staining step by placing a single layer of crystals of rhodopsin in a metal grid and imaging it with an electron microscope. Agard said that in this process, you see the atoms of the protein. This was a great improvement at the time, because at the time it was thought that it was impossible to resolve the protein structure using an electron microscope. Henderson et al. published this achievement in 1975.
In the 1980s and 1990s, the field of low temperature electron microscopy developed rapidly. A key breakthrough is the use of liquid ethane to rapidly freeze protein solutions. This is why it is called a cryo-electron microscope. But the resolution of this technology is only 1 nanometer, far from the need for drug design for protein structures. At that time, the resolution of X-ray crystal diffraction could reach 0.4 nm. Funders such as NIH have invested hundreds of millions of dollars to support the development of protein crystals, but there is very little funding for cryo-electron microscopy.
In 1997, Henderson attended the annual meeting of the 3D electron microscope at the Gordon Research Conference. A colleague made the opening speech with such words. "The low-temperature electron microscope technology is very limited, and it is impossible to surpass the X-ray crystal diffraction." But Henderson's idea is completely different. In the next speech, he made a counterattack. Henderson pointed out that cryo-electron microscopy will surpass other technologies and become the mainstream tool for studying protein structure worldwide.
The revolution begins
After that, Henderson et al. worked to improve the performance of electron microscopy—especially the sensitivity of the sensing electrons. Many years after digital cameras have been around the world, many electron microscopists still prefer to use traditional film because film can record electrons more efficiently than digital sensors. In collaboration with microscope manufacturers, researchers invented a new direct electron detector that is much more sensitive than film and digital camera detectors.
Around 2012, this detector was able to obtain continuous images of individual molecular atoms at high speeds of tens of frames per minute. At the same time, researchers like Scheres have carefully written software programs that build multiple 2D images into 3D models. The image quality of these 3D images is comparable to that obtained by X-ray crystal diffraction.
Low-temperature electron microscopy is useful for studying large, stable molecules that can withstand electron bombardment without distortion—a molecular machine made up of multiple proteins is the best sample. Therefore, the ribosome tightly surrounded by RNA is the best sample. Three chemist X-ray crystal diffraction studies of ribosomal solutions won the Nobel Prize in Chemistry in 2009, but these efforts took decades. In recent years, researchers in cryo-electron microscopy have also fallen into "ribosomal heat." Several teams have studied ribosomes in a variety of organisms, including the first high-definition model of human ribosomes. The results of X-ray crystal diffraction lag far behind LMB's Venki Ramakrishnan laboratory, and Venki won the 2009 Nobel Prize. Venki says that for large molecules, cryoelectron microscopy is far more practical than X-ray crystal diffraction.
In recent years, there have been many articles related to cryo-electron microscopy: in 2015, this technology was used for structural studies of more than 100 molecules. X-ray crystal diffraction can only image a single, static protein crystal, but cryo-electron microscopy can image multiple conformations of proteins, helping scientists infer the function of proteins.
In May, John Rubinstein, a structural biologist at the University of Toronto, used 100,000 low-temperature electron microscopy images to generate a "molecular film" of V-ATPase. The role of V-ATPase is to consume ATP and transport protons into it. The cell vacuole is shipped out. "We found that this enzyme is very flexible and can be bent, twisted and deformed," Rubinstein said. He believes that this is due to the flexibility of this enzyme, which efficiently transfers the energy released by ATP to the proton pump.
In 2013, the team of Nogales spliced ​​the structure of a complex that regulates the transcription of DNA into RNA. They found that a 10 nanometer structure tightly surrounding the DNA strand hangs on one arm of the complex, which may affect gene transcription. Nogales said that this structure is very beautiful, it can help us analyze the mechanism by which this molecule works.
Small and beautiful
Now that cryo-electron microscopy is developing rapidly, experts are looking for bigger challenges as the next analytical goal. For many people, the most wanted to resolve is the protein sandwiched in the cell membrane. These proteins are key molecules in the cellular signaling pathway and are also a relatively popular drug target. These proteins are difficult to crystallize, and cryoelectron microscopy is unlikely to image a single protein because it is difficult to extract these signals from background noise.
These difficulties cannot stop Cheng Yifan, a biophysicist at the University of California. He plans to resolve a small membrane protein TRPV1. TRPV1 is a receptor for detecting substances that cause burning sensation in peppers and is closely related to other pain-sensitive proteins. University of California pathologist David Julius and others tried to crystallize TRPV1 before failing. The TRPV1 project was analyzed with a cryo-electron microscope and progressed slowly at the beginning. However, at the end of 2013, technological advances made a major breakthrough in this project, and they obtained the structure of the TRPV1 protein with a resolution of 0.34 nm. The publication of this achievement is tantamount to thunder. This confirms that low temperature electron microscopy can resolve small, important molecules. "When I saw the structure of TRPV1, I was so excited that I couldn't sleep for a night," Rubinstein said.
Researchers may face more sleepless nights. Agard said that more membrane proteins will be resolved one after another.
An article published last September by Scheres and Tsinghua University's structural biologist Shi Yigong successfully resolved a membrane protein. They established a model of γ-secretase, which is responsible for the synthesis of β-amyloid plaques associated with Alzheimer's disease. The 0.34 nm resolution map shows that the relatively rare hereditary Alzheimer's disease γ-secretase mutation will present two “hot spots†on the map (mutations or sites with significantly increased recombination frequency), and this The mutation eventually synthesizes toxic beta-amyloid plaques. The structural map of γ-secretase helps researchers discover why previous inhibitors are ineffective and promotes the development of new drugs. Cheng Yifan said that the structure of γ-secretase is very amazing.
Similar success has attracted the attention of pharmaceutical companies. They hope to use a cryo-electron microscope to resolve proteins that cannot be crystallized, so that they can better develop drugs. Scheres is now working with Pfizer to overcome ion channels. Ion channels contain many membrane proteins, such as pain receptor molecules and neurotransmitter receptors. “I was almost contacted by everyone,†Nogales said.
Despite the rapid development of cryo-electron microscopy, many researchers believe that there is still much room for improvement. They hope to create more sensitive electronic detectors and better ways to prepare protein samples. In this way, smaller, more dynamic molecules can be imaged with higher resolution. In May, researchers published a structure of bacterial proteins with a resolution of 0.22 nm. This also shows the potential of cryogenic microscopes.
As with any popular field, the development of cryo-electron microscopy is also annoying. Some experts worry that researchers blindly pursuing the instrument will cause some problems. The structural map of HIV surface proteins in 2013 has been questioned by scientists who believe that many of the images used for modeling are white noise. Since then, X-ray crystal diffraction and cryo-electron microscopy models obtained by other teams have also questioned the original model. But these researchers insist on believing in their own results. In June of this year, at the Gordon Research Conference, researchers hoped that the structural drawings of cryo-electron microscopes would have strict quality control. And the magazine asked the authors to provide detailed modeling methods.
Cost issues may limit the promotion of cryo-electron microscopy. Scheres estimates that LMB's daily support for cryogenic electron microscopy amounts to nearly 30,000 RMB, plus nearly 10,000 electricity bills – because computers that store and process pictures consume a lot of power. Scheres said that it costs at least 40,000 yuan a day, which is too expensive for many places. In order to promote low-temperature electron microscopy, many foundations have established publicly available equipment, which can be reserved for use by researchers everywhere. The Howard Hughes Medical Institute (HHMI) is equipped with one at the Julia Farm Research Park. This device is open to researchers of all HHMI funds. In the UK, the government and the Wellcome Trust have established a platform for the use of cryo-electron microscopy near Oxford University. Helen Saibil, a structural biologist at the University of London who participated in the platform, said that many people want to learn to use cryo-electron microscopy.
One of these people is Rod MacKinnon, a biophysicist at Rockefeller University. He won the Nobel Prize in 2003 for analysing the crystal structure of some ion channels. MacKinnon is now fascinated by cryo-electron microscopy. “I am in the slope of the learning curve, very eager,†MacKinnon said. He plans to use a cryo-electron microscope to study how the ion channels are turned on and off.
In 1997, Henderson declared very firmly that cryo-electron microscopy would become a mainstream tool for analyzing protein structures. Today, nearly 20 years later, his predictions are more emboldened than in the past. Henderson said that if the low-temperature electron microscope keeps such momentum and the technical problems are solved, the low-temperature electron microscope will not only become the first choice for the analysis of protein structure, but the mainstream choice. This goal is not far from us.
Original search:
Ewen Callaway. (2015) The revolution will not be crystallized. Nature, 525 (7568): 172-174.
Office Staples,Staples Coupons,Staples Hyken,Staples Binders
SHAOXING YIYOU STATIONERY CO.,LTD , https://www.yiyou-staple.com